Infrared imaging in detection and evaluation of gaseous carbon dioxide
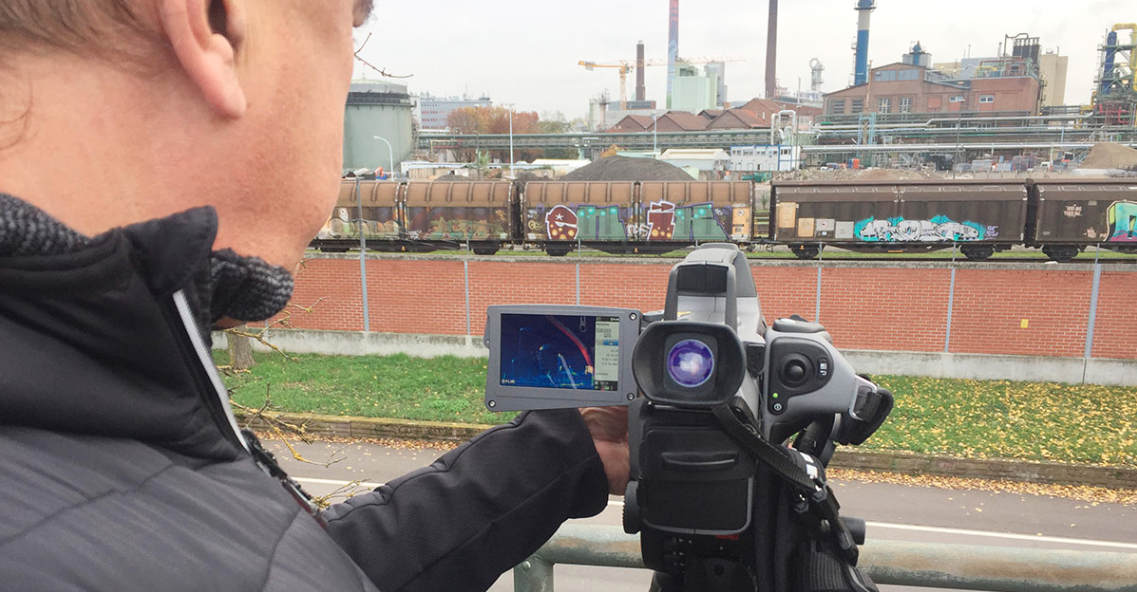
InfraMation 2018 Application Paper Submission
Jeehoon Choi and Jerry Seungyoun Kim
ABSTRACT
Gaseous carbon dioxide (CO2) is known as the main culprit of air pollution and is normally hard to detect because the energy emitted by gaseous CO2 is so small that it cannot be seen by the naked eye. On the one hand, an infrared imaging technique is viewed as one of the best potential solutions to quickly reveal invisible gaseous CO2. On the other hand, a guideline to visualize the CO2 leakage is needed for non-skilled inspectors since infrared imaging can detect only certain wavelengths depending on the properties of CO2. This paper presents the theoretical background and practical approaches to the detection and evaluation of CO2 leaks. Efforts are being made to analyze the presence of CO2 leaks in real problems.
INTRODUCTION
Gaseous CO2 accounts for less than 1 percent of atmospheric gases. Since the industrial revolution, however, the atmospheric levels of CO2 caused mainly by deforestation and burning of fossil fuels have risen. A delicate balance between CO2 and other gases are being disrupted and increasing CO2 leads to air pollution involved in the greenhouse effect, climate change, acid rain, and human health. Hence, a tremendous amount of work to restrain the increasing levels of CO2 has been implemented all over the world and various CO2 detection methods have been introduced.
The energy emitted by CO2 is so small that the localization of gaseous CO2 leaking from parts or equipment cannot be detected rapidly by the naked eye. However, infrared imaging makes it possible to not only visualize leaking CO2 in real time but also promptly pinpoint leak locations. This is because kinetic energy levels of gas molecules can be uniquely identified as spectral signatures in infrared absorption spectra. Oxygen molecules (O2), known as homonuclear molecules, do not absorb infrared radiation, while the vibration of CO2 molecules enables identification in the infrared spectral band. In other words, the major O2 component of the atmosphere is transparent in the spectral band, but CO2 is detected due to the infrared absorption. With the use of a band-pass spectral filter mounted in infrared cameras, leaking CO2 can be thus seen as a dark region against a higher temperature background. As reported by Reuters in October 2016, infrared imaging is the simplest but the best method to detect invisible CO2 [1].
Previous studies from the authors present gaseous methane (CH4) detection method with infrared camera having a detector sensitive to wavelength ranges between 3.2 and 3.4 μm. To be able to visualize the gaseous CH4 easily, both background temperature and emission were explained thoroughly and good contrast between CH4 and background was then emphasized [2]. The technique can be applied the same way to detect leaking CO2, but non-skilled inspectors found it most difficult. A guideline is thereby needed to effectively use the infrared imaging for leaking gaseous CO2. This paper describes guidelines based on theoretical background and practical approaches.
THEORETICAL BACKGROUND
Infrared radiation emanates from an object that emits energy from its internal molecular vibration. Figure 1(a) depicts the three types of CO2 molecules response to radiation. The arrows indicate the directions of motion. Let’s inspect the CO2 molecule vibration in the infrared spectral band. When CO2 molecules vibrate, the atoms move towards and away from each other at a certain frequency. As shown in Figure 1(b), two vibration modes, labeled (1) and (2), represent the stretching of the chemical bonds, one in a symmetric (1) fashion, in which both C=O bonds lengthen and contract together in-phase, and another in asymmetric (2) and (3) fashions, in which one bond shortens while the other lengthens.
It should be noted that the asymmetric stretch (2) and (3) are only infrared active because there is a change in the molecular dipole moment during the vibration. Otherwise, the symmetric stretch is not infrared active so that this vibration is not observed in the infrared spectrum of CO2. Figure 2 presents that the infrared radiation at 2349 cm-1 (4.26 µm) and 667 cm-1 (15 µm) excites the particular vibration (Note that wavenumber in x-axis is defined as the number of wavelengths per unit distance, typically cm-1.). The asymmetric stretch (2) causes nearly 100% absorption at 2349 cm-1 (4.26 µm), which divides the 3 to 5 µm window, namely mid-wave infrared (MWIR)
The spectral band is such a unique feature that it can serve as a signature to identify CO2 and be selectively visualized by means of a band-pass spectral filter for long-range observation and target recognition. The asymmetric stretch (3) causes a broad absorption band at 667 cm-1 (15 µm).
The transmittance at the particular wavelength depends on the concentration of CO2 and the optical path traveled and then this can be expressed as a formula, which is known as the Beer-Lambert law.
where, ππ(ππ, ππ) is the transmittance that relies on temperature (ππ) and wavelength (λ). πΌπΌ is the measured intensity after being absorbed by the medium, πΌπΌ0 is the source intensity on the medium and πΌπΌ(ππ, ππ) is the absorption coefficient, which depends on temperature and wavelength (can be found in the database of Reference [4] ). ππ refers to the concentration of CO2 and ππ is the absorption path length, which known as the optical path.
Infrared cameras can detect spectral wavelength from certain wavelengths of radiation that depends on surface temperature and surface characteristics of the object. The spectral wavelengths are converted to electrical signals amplified to create visible images. As shown in Figure 3, infrared cameras take images of infrared radiation emitted from surrounding, gaseous CO2, and background. The radiation originates from the surrounding and is reflected in background. The radiation from background and reflected radiation are influenced by the absorption of gaseous CO2 as well as radiation from gaseous CO2. Simultaneously, the absorption of atmosphere is also influenced. Each value is transmitted to a detector equipped in infrared camera.
A detection algorithm of the infrared camera to measure temperature assumes that the emissivity of the background is equal to unity based on a blackbody. Gaseous CO2 is considered as a gray body so that its emissivity is less than unity. The amount of radiation emitted by the gaseous CO2 relies on its emissivity that depends on temperature and optical path of the gaseous CO2. The absorption of radiation emanating from the gaseous CO2 leads to a reduction in radiation energy. Hence the gaseous CO2 is detected as a flickering cloud. To reduce the spectral range, the band-pass spectral filter of infrared camera is sensitive to only certain wavelength ranges between 3 and 5 μm.
Radiation energy terms are denoted in Figure 3. The total radiation energies measured using an infrared camera (πππ‘π‘π‘π‘π‘π‘) can derive a formula as follows, assuming that all of the radiation impinging on the surrounding at constant temperature (πππ π π π π π ) is absorbed by the same wall surface of the background (We can assume the emissivity of the surrounding is 1 on the basis of Kirchhoff’s law, πππ π π π π π =1). Here we also assume that the temperature of the wall surface (πππ€π€π€π€ππππ) and the temperature of gaseous CO2 (πππππ€π€π π ) are constant.
Four radiation energy terms can be defined as below:
The formula represents that a key parameter for the detection of gaseous CO2 is the contrast in transmitted radiation energies between CO2 and background. The detection thus occurs only if the background and gaseous CO2 are not in thermal equilibrium. If the temperature of gaseous CO2 is equal to the temperature of the background, gaseous CO2 is not able to be discriminated against the background. Therefore, inspectors strongly need to know how to make gaseous CO2 be distinguished from the background at given constraints. Practical approaches to detect and evaluate gaseous CO2 using infrared camera is outlined in the next section.
PRACTICAL APPROACHES
The FLIR GF-343 infrared camera has a spectral response from 4.52 to 4.67 μm, was selected in order to visualize gaseous CO2 in atmosphere. Figure 4 presents the rear images of vehicle when an engine just starts. As can be seen, the left image is a real picture and then any black smoke is not seen, while gaseous CO2 is seen at a glance from the right image taken by the infrared camera. Here it should be noted that the FLIR GF-343 does not support any thermal contrast control features but easily gets the shot. This is because the temperature of the gaseous CO2 is not equal to the temperature of exhaust pipe (called as “passive infrared imaging” that the features of interest are naturally at higher or lower temperature than surrounding.). Thus, inspectors can take infrared images right before the black smoke billows from the exhaust pipe of the vehicle.
The success of gaseous CO2 detection relies mainly on environmental conditions. The greater the background temperature difference is, the easier infrared cameras can visualize gaseous CO2 and pinpoint its source. While this indicates that gaseous CO2 can be detected by infrared camera anywhere, the specific amount of gaseous CO2 cannot be measured due to the environmental variants. Accordingly, inspectors need to use a portable measurement instrument near the gaseous CO2 point by point, a labor intensive and time-consuming process.
Let’s consider bad weather conditions such as strong wind and rain. As can be seen in Figure 5, gaseous CO2 clouds are detected when a car ran at full speed in the night. Due to good contrast results from temperature differences inspectors are able qualitatively to detect the gaseous CO2. It means that inspectors can see the clouds by creating a radiant contrast between the clouds and the background. The clouds itself reflect almost no radiation. Moreover, the wind helps visualize the clouds because it makes the gaseous CO2 move. Nevertheless, how can inspectors estimate quantitatively? One way to obtain the specific amount to estimate the distribution size of gaseous CO2 clouds from a database, hence predictive models on the basis of a pattern matching recognition process are should be studied to reduce unnecessary inspection time and expense. The authors’ ideas are described in detail on reference [2]. Rain makes detection very difficult. As shown in Figure 6, any clouds escaping through exhaust pipe are not seen in rain. This is because water absorbs over a wide range of radiation with rotational transitions and molecular vibrations responsible for absorption in infrared spectrum (~1 to 10 µm). So, visualizing gaseous CO2 in rainy weather is not recommended. Given that inspectors detect gaseous CO2 indoors, purging with dry air or nitrogen can be used to visualize gaseous CO2.
CONCLUSION AND OUTLOOK
Through infrared imaging guide based on brief theory and practices, non-skilled inspectors can not only learn how environmental conditions affect gaseous CO2 leak detection, but also visualize gaseous CO2 easily and pinpoint its source immediately. The use of infrared imaging is thus believed to be the most suitable method for fast detection and evaluation for real problems. The specific volume of leaks cannot be measured, but pattern recognition based on database information can help estimate it to reduce unnecessary inspection time and expense.
REFERENCES
[1] Reuters, “Infrared camera reveals 'invisible' air pollution”, October 10, 2016.
[2] Choi, J. and Kim, J. S., “A testing method development for detecting methane leakage based on
infrared optical imaging”, in: Proceedings of InfraMation 2016, Las Vegas NV, September 26-29, 2016.
[3] NIST Chemistry WebBook, https://webbook.nist.gov/cgi/
[4] Rothman, Laurence S., et al., "The HITRAN 2008 molecular spectroscopic database.", Journal of
Quantitative Spectroscopy and Radiative Transfer, Vol. 110, 2009, pp. 533-572.